Next: 5. Conclusions, some final
Up: 4. Fractal Strings
Previous: 4.1 The Hausdorf dimension
4.2 Classical-to-fractal geometric transition
The role of area resolution, as pointed out in the previous sections,
leads us to search for a critical area characterizing the transition
from classical to fractal geometry of the string stack.
To this end, we consider tha case in which the string possesses a non
vanishing average momentum, in which case we may use a
gaussian wave packet of the form (27).
The corresponding wave functional, in the
-representation, is
|
|
![$\displaystyle \Psi _{K} (C ; A)
=
\frac{[ (\Delta \sigma) ^{2} / 2 \pi] ^{3/4}}
{[ (\Delta \sigma) ^{2} + i A / 2 m ^{2}] ^{3/2}}
\cdot$](img128.gif) |
|
|
|
![$\displaystyle \quad \cdot
\exp
\left[
-
\frac{
\left(
\sigma ^{\mu \nu} \sigma ...
... \nu} / 4 m ^{2}
\right)
}
{2 [ (\Delta \sigma) ^{2} + i A / 2 m ^{2}]}
\right]$](img129.gif) |
(44) |
where we have used equation (34) to exchange
with
.
The corresponding probability density ``evolves'' as follows
|
|
![$\displaystyle \vert \Psi _{K} (C ; A) \vert ^{2}
=
\frac{(2 \pi) ^{-3/2}}
{
[
(\Delta \sigma) ^{2}
+
A ^{2} / 4 (\Delta\sigma) ^{2} m ^{4}
] ^{3/2}
}
\cdot$](img130.gif) |
|
|
|
![$\displaystyle \qquad \qquad \qquad \qquad \cdot
\exp
\left[
-
\frac{
\left(
\si...
...\sigma) ^{2}
+
A ^ {2} / 4 (\Delta \sigma) ^{2} m ^{4}
\right]}
\right]
\quad .$](img131.gif) |
(45) |
Therefore, the average area variation
,
when the loop wave packet drifts with a momentum
,
is
![\begin{displaymath}\langle \Delta S \rangle
\equiv
\left[
\int [ {\mathcal{D}...
...(C)
\vert \Psi _{K} (C ; \Delta A) \vert ^{2}
\right] ^{1/2}
\end{displaymath}](img132.gif) |
(46) |
For our purpose, there is no need to compute the exact
form of the mean value (46), but only its dependence on
.
This can be done in three steps:
- 1.
- introduce the adimensional integration variable
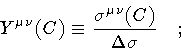 |
(47) |
- 2.
- shift the new integration variable as follows
 |
(48) |
- 3.
- rescale the integration variable as
![\begin{displaymath}\bar{Y}^{\mu \nu} (C)
\rightarrow
Z ^{\mu \nu} (C)
\equiv
...
...) ^{2}}{4 m ^{4} (\Delta \sigma) ^4}
\right] ^{1/2}
\quad .
\end{displaymath}](img135.gif) |
(49) |
Then, we obtain
where,
The parameter
measures the ratio of the ``temporal'' to ``spatial''
uncertainty, while the area
sets the scale of the
surface variation at which the string momentum is
.
Therefore, always with the particle analogy in mind,
we shall call
the loop DeBroglie area.
Let us assume, for the moment, that
is
independent of
,
so that either quantity can be
treated as a free parameter in the theory. A notable exception to this
hypothesis will be discussed shortly. Presently, we note that
taking the limit
,
affects only the first
term of the integral (50), and that its weight with respect
to the second term is measured by the ratio
.
If the area resolution is much
larger than the loop DeBroglie area, then the first term is negligible:
is independent of
and
scales as
 |
(53) |
In this case, independence of
is achieved by
assigning
D H = 2.
As one might have anticipated, the detecting apparatus is
unable to resolve the graininess of the string stack, which
therefore appears as a smooth two dimensional surface.
The fractal, or quantum, behavior manifests itself below
,
when the first term in (50) provides the
leading contribution
 |
(54) |
This expression is less transparent than the relation (53),
as it involves also the
resolution. However, one may now consider
two special subcases in which the Hausdorff dimension can be
assigned a definite value.
In the first case, we keep
fixed, and scale
down to zero. Then, each
diverges,
because of larger and larger shape fluctuations, and
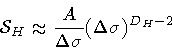 |
(55) |
requires
D H = 3.
The same result can be obtained also in the second subcase, in
which both
and
scale down to zero,
but in such a way that their ratio remains constant,
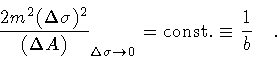 |
(56) |
The total interior area
is kept fixed. Therefore, as
,
then
in order to keep A finite. Then,
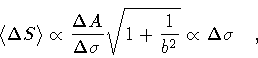 |
(57) |
and
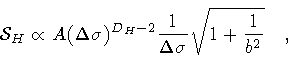 |
(58) |
which leads to
D H = 3 again. In the language of fractal
geometry, this interesting subcase corresponds to
self-similarity. Thus, the condition (57) defines
a special class of self-similar loops characterized by
an average area variation which is proportional to
at any scale.
Next: 5. Conclusions, some final
Up: 4. Fractal Strings
Previous: 4.1 The Hausdorf dimension
Stefano Ansoldi
Department of Theoretical Physics
University of Trieste
TRIESTE - ITALY